Distinguished alumnus and donor Paul Cadario (CivE 7T3, Hon LLD 1T3) has had a significant impact on the lives of thousands of students.
His passion for education and community development is reflected through his generous contributions to student scholarships and university infrastructure. His transformational gifts include establishing the Paul Cadario Chair in Global Engineering, the Paul Cadario Civil Engineering Award, and the Experiential Learning Student Awards and Social Impact Internships in both the Faculty of Arts & Science and the Faculty of Applied Science & Engineering.
For U of T Giving Day — a 24-hour fundraising campaign on March 25 — writer Kristina Kazandjian spoke with Cadario about his journey from civil engineering to the World Bank, the impact of scholarships on his education, and the importance of giving back.
What are your thoughts on engineering as a profession that can lead you anywhere?
Engineers are trained to see systems and operate within complex environments. These skills are invaluable in a wide range of fields that engineers take up with great success, from technology to law to business.
For example, my own field, global development and poverty eradication, involves intricate economic, financial and political systems that evolve rapidly and sometimes unpredictably.
The adaptability that an engineering education provides makes it a great way to open doors to countless opportunities.
What was the first donation you made to U of T and what was the inspiration behind the gift?
The first donation I remember was to support a modest scholarship; as a young World Bank staffer with a mortgage, it was all I could scrape together at the time. But it felt important to do so. It was my way of saying thank you for the U of T entrance scholarship, and for the Inco scholarship that covered my tuition.
This financial aid meant that my mother, who was widowed young, didn’t have to worry about supporting me through university. The scholarships I received meant I could work summer jobs related to engineering, gaining practical skills before graduation. Measuring water currents and temperatures at future Ontario Hydro generating sites and examining municipal services in Inuit communities in Nunavut gave me real-world insights into how engineering impacts communities.
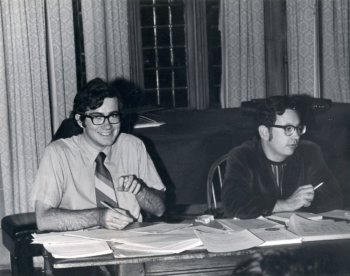
You chose a somewhat unconventional career path after engineering: the World Bank. How did your time there shape your approach to philanthropy and giving back?
It’s fair to say that graduating from civil engineering, specialized in urban transport and the environment made me close to being a social scientist and comfortable with quantitative economics and data analysis. This skill set was invaluable when I joined the World Bank, which still hired many engineers due to its origins in reconstruction and development.
For example, my training helped me explain to colleagues why groundwater irrigation on the Greek side of a river would impact groundwater on the Turkish side as well, and the political problems that might raise. Another example: an architect colleague and I concluded that a government’s interest in an urban freeway had more to do with who owned the property than it did with inflated traffic counts. The hours I’d spent counting cars turning from McCaul onto College Street during Traffic Engineering and analyzing the number of homes the Scarborough Expressway would cut through taught me to consider the broader impact of investments on real people.
Ultimately, philanthropy is about making a tangible difference in people’s lives, whether through better classrooms and labs for students or ensuring that the brightest minds have access to education regardless of their financial means.
You’ve made significant contributions to the University of Toronto, mostly to student awards. What motivates your generosity toward the university and why is student access so important?
I owe my education to the generosity of others, having attended U of T and Oxford on scholarships. Giving back is my way of paying it forward and ensuring that talented students can attend U of T regardless of their financial situation.
On top of that, my volunteer work since graduating has given me a deep understanding of all the incredible teaching and research happening at U of T. I want to support, maintain and expand that excellence.
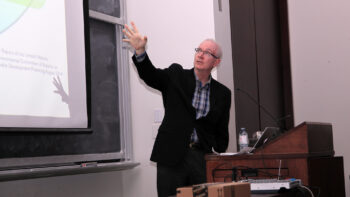
How do you see your philanthropic efforts benefiting the next generation of students and the broader community?
Our future depends on having great scholars and teachers advancing knowledge. I’m thrilled with the impact the Centre for Global Engineering has had, from bringing water innovation to poor communities to advancing rapid diagnostics for health centres.
Graduates from U of T Engineering and the Munk School have gone on to influential roles in government, consulting, banking and other sectors, reflecting well on U of T’s academic community. Investments in facilities for the Department of Civil & Mineral Engineering and University College create spaces for learning and discussion. Bringing people together in this way is what great research universities do.
Can you share a story or moment that made you especially proud of your involvement with the university?
There are many moments, but celebrating Geoff Hinton’s Nobel Prize in Stockholm stands out.
His work on artificial intelligence has had a profound impact on science and our daily lives, highlighting the importance of addressing the ethical and societal implications of technological advancements. It’s moments like these that make me proud to support U of T.
For someone interested in giving back but unsure where to start, what would you recommend as the first step?
Volunteer. Learn about what’s happening at U of T in a field that excites you and talk to a favourite professor about how you can get involved.
Engage with your community and share how U of T and other post-secondary institutions contribute to society. Encourage young people to consider U of T for their education and future careers.
On March 13, U of T Engineering students were celebrated for their leadership and service to the university at an event hosted by the U of T Engineering Office of Advancement, the Engineering Society (EngSoc), and the offices of the Vice-Dean Undergraduate and the Vice-Dean Graduate.
At the celebration, 16 students were presented with the University of Toronto Student Leadership Awards (UTSLA), recognizing their contributions to student clubs and design teams, fostering inclusivity through outreach activities and events, and advocating for students by strengthening support systems for academic success, mental health and sexual violence prevention.
The UTSLA was established in 1994 by the University of Toronto Alumni Association in honour of Gordon Cressy, former vice-president, development and university relations to celebrate students whose service has had a lasting impact on their peers and the university.
Also at the event, members of EngSoc and the Graduate Engineering Council of Students (GECoS) celebrated their outgoing student leaders, and recognized the contributions made to student life with the EngSoc Awards.
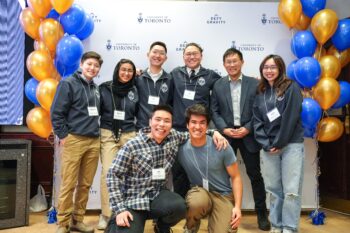
“Our student leaders work tirelessly each year to make our community a better place for everyone. Their contributions inspire their peers, as well as future students, and make this faculty such a wonderful place,” says Chris Yip, Dean of U of T Engineering.
“I look forward to seeing what each of them will accomplish as our future engineering leaders. Congratulations to all our U of T Engineering student leaders.”
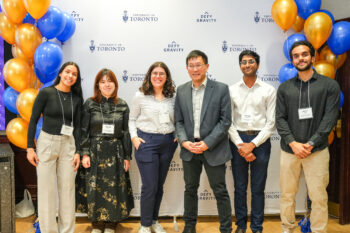
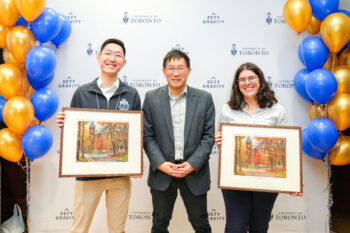
The UTSLA recipients for 2025 are:
- Abeer Fatima
- Armita Khashayardoost
- Ashna Jain
- Badr Abbas
- Cassidy Tan
- Dina Aynalem
- Janishan Jeyarajah
- Madeline Kalda
- Prarthona Paul Priya Jadav
- Seung Jae Yang
- Sophie Sun
- Tobin Zheng
- Vivek Vinay Dhande
- Wanda Janaeska
- Zijie (Jason) Zhou
Outgoing EngSoc Leadership
- Inho Kim, President
- Zayneb Hussain, Vice-President of Finance
- Jennifer Wu, Vice-President of Communications
- Katherine Jia, Vice-President of Academics
- Sean Huang, Vice-President of Student Life
Outgoing GECoS Leadership
- Tess Seip, President
- Brohath Amrithraj, Vice President of Finance
- Melody Li, Vice President of Communications
- Sharini Sam Chee, Vice President of Student Life
- Aryan Singh, Vice President of Professional Development
EngSoc Award Winners
- Engineering Society Centennial Award: Justin Fang and Christopher Lee
- Engineering Society Semi-Centennial Award: Lauren Altomare and Kai Hashimoto
- Engineering Society Award: Sean Huang
- Skule™ Cannon Award: Ashna Jain
- Discipline Club of the Year: Mechanical Engineering Club
- Affiliated Club of the Year: University of Toronto Nuclear Energy Association
- Director of the Year: Tait Berlette and Zijie (Jason) Zhou
- Representative of the Year: Kelvin Lo and Sebastian Kiernan
- Joe Club Award: Badr Abbas
- L.E. Jones Award for Arts in Engineering: Reid Sox-Harris
See more photos from the event in our Flickr gallery.
Researchers at U of T Engineering, led by Professor Yu Zou (MSE), are leveraging machine learning to improve additive manufacturing, also commonly known as 3D printing.
In a new paper, published in the journal of Additive Manufacturing, the team introduces a new framework they’ve dubbed the Accurate Inverse process optimization framework in laser Directed Energy Deposition (AIDED).
The new AIDED framework optimizes laser 3D printing to enhance the accuracy and robustness of the finished product. This advancement aims to produce higher quality metal parts for industries, such as aerospace, automotive, nuclear and health care, by predicting how the metal will melt and solidify to find optimal printing conditions.
“The wider adoption of directed energy deposition — a major metal 3D printing technology — is currently hindered by the high cost of finding optimal process parameters through trial and error,” says Xiao Shang (MSE PhD candidate), first author of the new study.
“Our framework quickly identifies the optimal process parameters for various applications based on industry needs.”
Metal additive manufacturing uses a high-powered laser to selectively fuse fine metallic powder, building parts layer by layer from a precise 3D digital model.
Unlike traditional methods, which involve cutting, casting or machining materials, metal additive manufacturing directly creates complex, highly customized components with minimal material waste.
“One major challenge of 3D metal printing is the speed and precision of the manufacturing process,” says Zou. “Variations in printing conditions can lead to inconsistencies in the quality of the final product, making it difficult to meet industry standards for reliability and safety.
“Another major challenge is determining the optimal settings for printing different materials and parts. Each material — whether it’s titanium for aerospace and medical applications or stainless steel for the nuclear reactors — has unique properties that require specific laser power, scanning speed and temperature conditions. Finding the right combination of these parameters across a vast range of process parameters is a complex and time-consuming task.”
These challenges inspired Zou and his lab group to develop their new framework. AIDED operates in a closed-loop system where a genetic algorithm — a method that mimics natural selection to find optimal solutions — first suggests process parameters combinations, which machine learning models then evaluate for printing quality.
The genetic algorithm checks these predictions for optimality, repeating the process until the best parameters are found.
“We have demonstrated that our framework can identify optimal process parameters from customizable objectives in as little as one hour, and it accurately predicts geometries from process parameters,” says Shang. “It is also versatile and can be used with various materials.”
To develop the framework, the researchers conducted numerous experiments to collect their vast datasets. This essential but time-consuming challenge ensured that the datasets covered a wide range of process parameters.
Looking ahead, the team is working to develop an enhanced autonomous, or self-driving, additive manufacturing system that operates with minimal human intervention, similar to how autonomous vehicles drive themselves, says Zou.
“By combining cutting-edge additive manufacturing methods with artificial intelligence, we aim to create a novel closed loop controlled self-driving laser system,” he says.
“This system will be capable of sensing potential defects in real-time, predicting issues before they occur, and automatically adjusting processing parameters to ensure high-quality production. It will be versatile enough to work with different materials and part geometries, making it a game-changer for manufacturing industries.”
In the meantime, the researchers hope AIDED will transform process optimization in industries that use metal 3D printing.
“Industries such as aerospace, biomedical, automotive, nuclear and more would welcome such a low-cost yet accurate solution to facilitate their transition from traditional manufacturing to 3D printing,” says Shang.
“By the year 2030, additive manufacturing is expected to reshape manufacturing across multiple high-precision industries,” adds Zou. “The ability to adaptively correct defects and optimize parameters will accelerate its adoption.”
Mohammadamir (Amir) G. Moghaddam (BME PhD student) has been named the recipient of the 2025-26 Adel S. Sedra Distinguished Graduate Award, recognizing his outstanding academic achievements and leadership. The award, established in honour of former U of T vice-president and provost, Professor Adel S. Sedra (ECE), is given annually to a doctoral student who exemplifies excellence in research and extracurricular leadership in university and community activities. It is administered by the University of Toronto Alumni Association (UTAA).
Moghaddam’s research focuses on Polymer-DNA origami hybrid cancer vaccines. His work has been published in Advanced Drug Delivery Reviews and Biofabrication, and has received over $300,000 in competitive funding and scholarships. He is a Vanier Scholar and a Connaught PhDs for Public Impact Fellow.
In addition to his research, Moghaddam has held leadership roles in student governance. As president of the University of Toronto Graduate Students’ Union (UTGSU), he was involved in securing a university-wide increase in PhD/SJD funding to $40,000 annually and implementing programs addressing food insecurity. He also contributed to initiatives exploring the establishment of a non-profit housing corporation for graduate students.
Moghaddam’s advocacy extends beyond the university. As chair of the Canadian Federation of Students-Ontario Graduate Students Caucus and the CFS International Students Constituency, he has represented over 80,000 graduate students across Ontario, contributing to policy discussions on student funding and discounts. He has also worked on programs aimed at connecting graduate researchers with cancer patients and high school students. Under his leadership, UTGSU introduced more than 20 new graduate student employment positions.
Zayne Thawer (EngSci 2T0+ PEY) never imagined the career path his Professional Experience Year Co-op Program (PEY Co-op) at Safran Landing Systems would open up.
“My internship was incredibly enlightening. It introduced me to the world of industry beyond academia,” he says.
“As a systems engineering and architecture intern, I worked with a team of engineers in Canada and France on the development and implementation of a Model-based Systems Engineering (MBSE) framework using the Systems Modeling Language (SysML). This modeling was derived from the NASA Systems Engineering Handbook and was done for the landing gear architecture and operation of a supersonic jet program.”
Today he is driven by a passion for creating a positive impact on society. As a spacecraft systems engineer at Planet Labs — a satellite imagery and earth data analytics company based in San Francisco — he is dedicated to advancing space sustainability. His work ensures that space remains a viable resource for future generations.
“One area of innovation that I’ve been able to work on is the art of maneuver automation. As space becomes an increasingly dense environment with lots of satellites, debris and objects of all kinds, we are making sure that we are operating in a safe and sustainable manner,” he says.
“Prior to my time at Safran, I did not understand what the field of systems engineering was, and now I work as a space systems engineer on a constellation of Earth-orbiting satellites.”
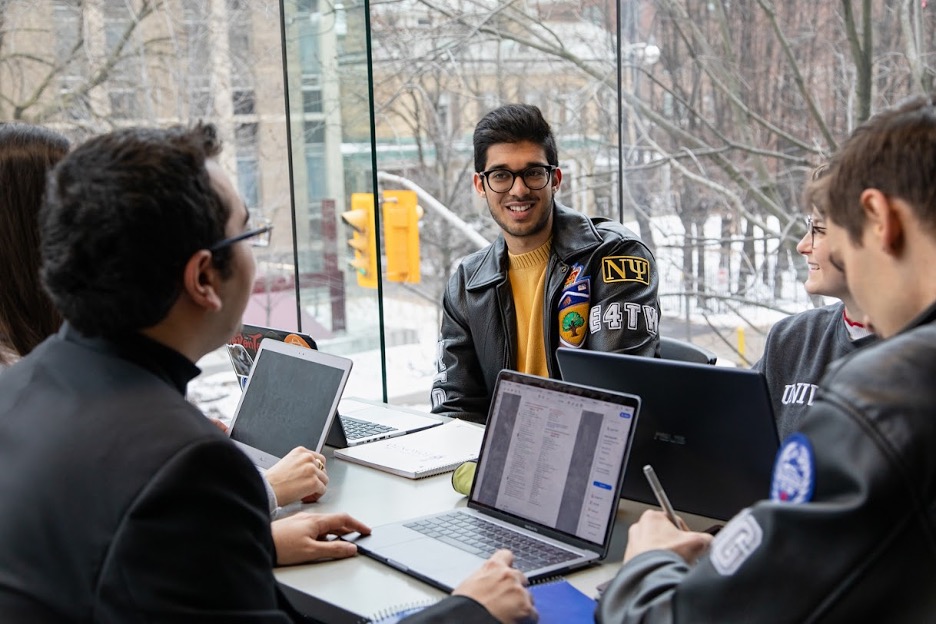
The PEY Co-op program, offered through the Engineering Career Centre, is a work-integrated learning initiative designed to help U of T Engineering undergraduate students gain paid, meaningful work experience aligned with their individual career goals. With up to 20 months of work experience, PEY Co-op students can engage in long-term projects from inception to completion; while earning a competitive salary and developing a robust professional network and valuable skills they can apply throughout their careers.
Thawer’s PEY Co-op experience gave him the opportunity to work on different projects that enabled him to gain new capabilities. During his time at Safran Landing Systems, Thawer tracked change management for the Bombardier Global 7500 aircraft program, through which he learned how to write and manage requirements, as well as capture validation and verification activities of a broad range of subsystems and components.
Two key skills he honed during his time at Safran Landing Systems were context switching and being a high-level systems thinker.
“The first project I did at Safran Landing Systems had a profound impact on my career trajectory, as it was the first time I was faced with an ambiguous, high-level problem that needed to be broken down into manageable pieces using critical thinking and intuition,” says Thawer.
“This systems-thinking approach to a challenge that did not have one correct answer was extremely rewarding to work on, since it forced me to think creatively about a deeply interconnected system and untangle the complexity,” he says.
“In my current role at Planet, working on the Mission Operations team, there’s always a million things going on at the same time and being able to jump from one project to the next is a skill that I started working on when I was at my PEY Co-op at Safran Landing Systems. It also was a big part of the Engineering Science curriculum.”
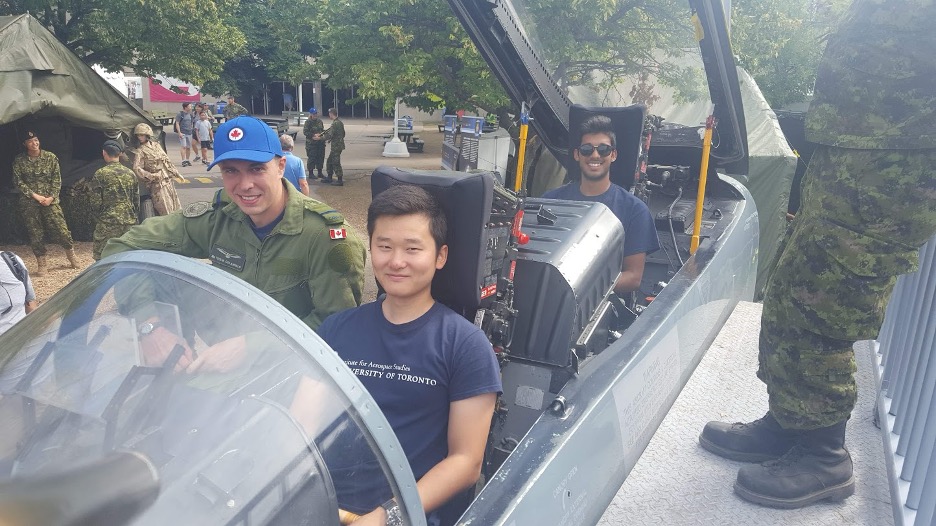
As an advocate for space sustainability, Thawer feels fortunate to contribute to systems that account for satellite manoeuvres and fleet management to reduce the risk of collisions in space.
“We have developed systems that proactively identify and mitigate collisions that may be imminent in the coming days,” he says. “I think space sustainability is going to become an increasing concern as we see the rise of the commercial space industry. It’s important that we all consider the impact that our actions are having on the future of the space environment.”
For students embarking on their PEY Co-op journey, Thawer’s advice is to step out of their comfort zones and embrace new opportunities.
“Without the PEY Co-op program’s on-the-job training to develop my skills in analyzing nebulous, multi-disciplinary engineering problems, I would not be on the path I currently tread. But every opportunity I could get to work with another team or project broadened my horizons,” he says.
Looking ahead, Thawer is eager to deepen his expertise in the space industry.
“I think there’s still so much more for me to learn about spacecraft design and operations. I’m looking forward to taking on new technical challenges and becoming a well-rounded engineer at Planet,” he says.
When Laura Thompson (ChemE 1T6), was in her fourth year at U of T Engineering, a simple skincare hack sparked her journey into the world of natural skincare.
“One of my friends introduced me to using coconut oil as a natural makeup remover,” says Thompson, co-founder of Three Ships. “I loved how well it worked, but I found the user experience of scooping it out of a jar and applying it to my face to be bulky and cumbersome.”
Thompson started to research more natural skincare alternatives but was frustrated with companies making questionable claims about being natural and clean — a practice sometimes referred to as greenwashing — as well as a general lack of clarity and transparency around these terms.
“Three Ships was born out of our own frustration,” says Thompson, who, along with her co-founder Connie Lo, invested $2,000 into the creation of the company. “We started by hand-making our products in our kitchen. Our intention was to create truly natural skincare products that are affordable and deliver exceptional results.”
Today, Three Ships no longer makes its products in the co-founders’ kitchen. The company works with three factories in the Greater Toronto Area — and one in South Korea for their innovative biodegradable eye masks — to deliver mostly made-in-Canada products.
“One of the big advantages is that we’re able to have boots on the ground and go and visit our contract manufacturers to ensure quality control,” says Thompson.
“That’s why all our products that we can make locally are made locally. It was a huge decision for us to even start making products in South Korea, but there were no contract manufacturers here that could make that type of technology.”
For Sofia Bonilla (ChemE PhD 1T7), establishing a Canadian company with made-in-Canada products has been a positive experience due to the support she has received, including from U of T Mississauga’s ICUBE — a social entrepreneurship accelerator for early-stage startups.
“It’s incredibly difficult to build a business, so I won’t sugar coat it, but there have been so many people wanting to help us and see us succeed,” she says.
Bonilla is the founder and CEO of ALT-PRO Advantage, makers of next generation pet foods that use environmentally friendly, nutrient-rich insect protein.
Before becoming an entrepreneur, Bonilla spent her career in academia. Born and raised in Colombia, she completed a degree in microbiology before moving to Australia to complete a master’s degree in environmental science.
She then completed her PhD in chemical engineering at U of T and pursued postdoctoral fellowships in Toronto and the Netherlands.
“My expertise is in protein. For many years, I was thinking about protein, learning about protein and over time I came to understand the importance of diversifying where we get our protein,” she says.
“Combining my biology background with engineering helped me develop an understanding of manufacturing products. I realized I could use my scientific training to create products that people could buy, helping them transition to environmentally friendly, nutritionally equivalent alternative protein sources.”
ALT-PRO started using insect protein in pet foods due to its commercial availability, as well as its proven safety, palatability and digestibility.
“We see a lot of opportunity in other alternative proteins, and we are even developing our own ingredients that we plan to incorporate in the future using microalgae. We have these cool, proprietary ingredients that will be a source of essential nutrients for pets but also has different applications in other feed and food products,” says Bonilla.
“The company started with just me, but we are now a team of six with research and development based in Halifax. We also have products in 84 stores across Canada.”
Both ALT-PRO Advantage and Three Ships are Certified B Corporations, a designation from nonprofit network B Lab, which indicates that a company meets the highest standards in social and environmental performance, public transparency and legal accountability.
Building a business in Canada has its challenges, says Thompson, noting that accessing capital has been one of Three Ships’ biggest hurdles, especially since many investors and large venture capitalist firms are based in the United States.
But the company is now at the stage where it has a lot of Canadian visibility, she says. Their next goal is to continue expanding and get their products into a major beauty retailer.
“My biggest advice for women in engineering who are interested in pursuing entrepreneurship is to learn how to sell,” she says. “As a founder, you are always selling something: the culture of your team to new employees, the future growth potential to investors and your products to your customers and retail buyers.
“I wish that more women would go into a sales role right out of school and learn those skills before launching their companies. Connie and I both had sales backgrounds before we started, and we learned so much from those experiences, which serve us in entrepreneurship.”
If Bonilla could give her past self one piece of advice, it would be to have more confidence in her vision early on.
“I’m learning to not undersell accomplishments,” she says. “My advice to women interested in entrepreneurship is to trust and surround yourself with people who believe in you and your vision. Don’t be afraid to be authentic and change the way things have been traditionally done.”