This article originally appeared in the 2016 issue of Skulematters magazine.
Interdisciplinary research from U of T Engineering is helping us live longer, healthier lives. These projects from across our Faculty illustrate leading-edge innovations that will improve health care, from planning and prevention to diagnosis and treatment:
Optimizing surgical schedules

Long wait lists for elective surgeries are a major challenge in the Canadian health care system. According to Professor Dionne Aleman (MIE), pictured below, right, the problem may not necessarily be a lack of resources, but rather a result of not using the resources we have as efficiently as we could.
“Hospitals have rules that indicate who gets operated on when, but the schedules that result are sub-optimal,” she said. Aleman and her team are addressing this problem by building mathematical models that can optimize the matches between patients, surgeons and operating rooms to generate the most efficient schedule.
One technique the team uses involves pooling resources. Rather than each hospital maintaining its own waiting list, patients would be treated as a single large waiting list. Patients would be assigned to a given surgeon or operating room to minimize the time when resources are unused. Using mathematical optimization tools, the team has generated schedules that could increase the number of patients treated in a given time period by up to 30 per cent.
Aleman collaborates closely with Dr. David Urbach, a surgeon and senior scientist at the Toronto General Research Institute. Her models are based on data from Toronto General Hospital, Toronto Western Hospital and the Princess Margaret Cancer Centre.
“Right now we’re adding workload balancing into our models,” Aleman said. “We want to ensure that all surgeons and hospitals are being used to an equitable level.”
Some hospitals are already using patient pooling on a small scale, and Aleman hopes that her models will help the technique be applied more broadly.
“I like research that has a positive impact on the world,” she said. “In health care it’s very obvious to see how any improvements can have a wide-ranging impact, so it’s a very fulfilling type of work to do.”
Walk this way
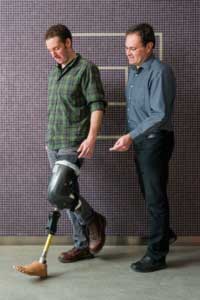
Jan Andrysek’s research helps amputees walk tall. A professor at IBBME and scientist at the Bloorview Research Institute in the Holland Bloorview Kids Rehabilitation Hospital, he is the engineer behind the AT-Knee (short for “all-terrain”) — a mechanical prosthetic knee joint that is natural feeling, durable, energy-efficient and inexpensive.
“Most affordable prosthetic knees rely on mechanisms that are not designed to concurrently provide safe and efficient mobility, so they often feel awkward and unstable during walking,” said Andrysek, pictured above, right. “The AT-Knee is designed to lock and unlock smoothly as you walk, and move just like the human knee joint.”
While typical prosthetic knees can carry price tags of $1,000 to $50,000, the AT-Knee is available at a much lower cost through NGOs, making it a huge step forward for patients with lower-limb impairments, especially in war-torn or developing nations. His team has tested their design in over 15 countries around the world. In April 2014, Andrysek along with three co-founders launched the company LegWorks to commercialize the AT-Knee technology. As of January 2016, the AT-Knee is commercially available in many countries globally.
Andrysek and his research group are now working to improve communication between people’s bodies and their artificial limbs. His lab is designing a non-invasive biofeedback system to improve the wearer’s awareness of limb position.
“Basically, using small vibratory signals, the system provides amputees with information about the position and loading of the artificial limb to improve mobility,” he said.
Human bodies elegantly integrate chemical, electrical, materials and mechanical systems — and Andrysek’s research is just as multidisciplinary. In addition to colleagues across the University of Toronto and hospital networks, he works directly with prosthetics companies, non-governmental organizations and clinical partners around the globe.
“These collaborations help us define important areas of need, and subsequently help us deliver our solutions to children and adults who stand to benefit from them most,” Andrysek said.
Optimizing ambulance distribution and routing
In Toronto, the average response time for ambulances is six minutes. In Dhaka, Bangladesh, the 11th largest city in the world, it’s 60 to 80 minutes. Professor Timothy Chan (MIE) and his team are working to help close this health-services gap.
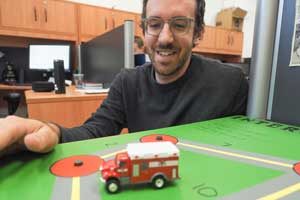
“Our research has two components: first, optimizing the locations of ambulances throughout the city, and second, routing them to the patients,” said Justin Boutilier, a PhD candidate in Chan’s lab.
In September 2015, Boutilier spent three weeks in Dhaka assessing the current situation. Collaborating with Moinul Hossain, a traffic engineer and professor from the Islamic University of Technology (IUT), Boutilier surveyed patients regarding emergency transportation to hospitals to better understand the current state of the system. He also distributed a GPS-based app to rental car drivers that will track driving speeds and help map traffic patterns in the city.
All this information will be fed into a computer optimization model, which will test out hundreds of possible scenarios before arriving at an ideal distribution of ambulances across the city. The data will also be used to develop an app that provides real-time recommendations to drivers on the fastest route. In a city where it is not the cultural norm to yield to ambulances — and where there is little room to move aside anyway — avoiding busy streets can mean the difference between life and death.
Boutilier and Chan will also evaluate other forms of transportation, such as three-wheeled cabs or rickshaws, in order to address issues with reaching patients in areas without adequate access to ambulance transport, such as slums. They hope their approach can bring significant health benefits for the citizens of developing countries.
“There is a long history of using operations research to improve emergency medical services in North America, but in developing countries many of these ideas are still very new,” Chan said. “I think we can make a big impact there.”
Reducing the risks of cycling

Cycling and walking are good for your health, right? It’s actually a more complicated question: while increased physical activity can improve cardiovascular function, cyclists and pedestrians are also exposed to air pollution, which can do the opposite. Professor and alumna Marianne Hatzopoulou (CivE) and her team aim to help city dwellers get more of the benefits of physical activity while reducing the risks.
This summer, Hatzopoulou and her team collaborated with Health Canada on a study that aimed to map out how pollution affects human health in different areas of the city. Volunteers walked the streets of Toronto equipped with GPS devices and instruments to monitor their heart rates and blood pressure, as well as the level of noise and air pollution they are exposed to. By correlating location with pollution and physiological data, the team can find the cleanest, safest routes.
Hatzopoulou has already created an online tool that does just that, based on the results of previous studies. The Clean Ride Mapper leverages Google Maps to help cyclists find routes that have lower levels of pollutants like ultra-fine particles or nitrogen oxides.
The next step is to determine how cyclists weigh the value of reduced pollution exposure against other factors involved in choosing a route, including separation from traffic, elevation and distance. Hatzopoulou and postdoctoral researcher Sabreena Anowar (CivE) are measuring this through an online survey launched this past June.
While no route is perfect, Hatzopoulou said that for most people, the benefits of physical activity outweigh the risks.
“If you are a healthy person, you are better off to continue cycling or walking than stop,” she said.
Hatzopoulou hopes that her research will help city planners create walking or cycling infrastructure and is both safe and clean, making life in cities that much healthier.
Target practice on tumors

The side effects of cancer treatment can be painful: hair loss, ulcers and nausea. Cancer drugs cause damage because they destroy fast-growing cells indiscriminately, whether they’re cancerous or healthy. Professor Warren Chan (IBBME), pictured above, right, has spent the last decade figuring out how to deliver chemotherapy drugs directly into tumours — and nowhere else.
Chan, a pioneer in the field of nanomedicine, brings a holistic view to his research. His group works on engineering more effective nanoparticles for drug delivery, modelling and imaging the behaviour of nanoparticles inside the body, and studying the effectiveness — or ineffectiveness — of nanoparticle treatments. The group published a paper in spring 2016 revealing that less than one per cent of nanomedicines reach their intended tumour targets. Now they’re trying to find out why.
“We felt it was time to look at the field more closely,” Chan said. “The amount of research into using engineered nanoparticles to deliver cancer drugs directly to tumours has been growing steadily over the last decade, but there are very few formulations used in patients. Our paper illuminated some major limitations we’re now working to overcome.”
Most of the nanoparticles get stuck in the liver, spleen and kidneys — organs responsible for filtering toxins and waste from blood. Chan’s group is now examining how the liver takes up nanoparticles, in partnership with Dr. Ian McGilvray at Toronto General Hospital, Professor Anton Zilman in the Department of Physics, and Professor Julie Audet in IBBME.
“Our connections to the rest of the University, as well as the surrounding research hospitals, really help us drive our research forward,” Chan said. “Our best work happens when we’re looking at the problem from multiple angles.”
Lab-grown tissues could help repair damaged hearts
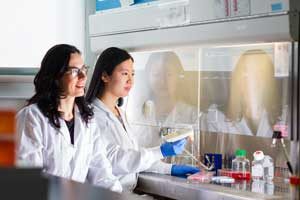
Professor Milica Radisic (IBBME, ChemE) and her team create sophisticated, realistic systems for growing human cells outside the body. Their lab-grown tissues are already being used to discover and test new drugs, and could one day replace or repair damaged organs.
The team’s latest invention, the AngioChip, is a three-dimensional scaffold complete with tiny channels that act as internal blood vessels. Made of POMaC, a polymer that is both biodegradable and biocompatible, the AngioChip is seeded with heart or liver cells, which attach to the surface and begin growing just like they would in the body.
“Our heart muscle tissue actually beats, and our liver produces urea and metabolizes drugs,” said Radisic.
The team has licensed the technology to a spinoff company, TARA Biosystems, which uses the lab-grown tissues to screen new drug candidate molecules for negative side effects on the heart or liver. This reduces the use of animal testing and can prevent potentially dangerous drugs from reaching the market.
In the future, the tissues could also be used to screen large libraries of molecules for beneficial effects, helping to discover new drugs. But the ultimate goal would be to implant the lab-grown tissues back into patients whose own organs have become damaged through disease or injury.
Radisic and her team have many collaborators, from fellow U of T professors Michael Sefton (ChemE, IBBME) and Aaron Wheeler (Chemistry, IBBME), to researchers at the University Health Network and her business partners at TARA Biosystems.
“It’s all about meeting the right people and making connections,” she said. “When you are able to look back and see something you invented in lab turn into something that could really help people, I think that’s very motivating.”
Mind reader
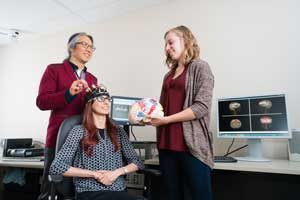
Professor Tom Chau (IBBME) wants to break down communication barriers. He has devoted his career to applying engineering solutions to clinical settings, with the goal of helping children with disabilities share their opinions and reach their goals with their families and in daily activities of life.
Chau, who is also the vice-president of research at Holland Bloorview Kids Rehabilitation Hospital, is working to find non-invasive ways for children with limited mobility, motor control or loss of speech to communicate and interact with the world around them using technology. One of his latest projects is an intuitive brain-computer interface that uses near-infrared spectroscopy to image blood flow on the surface of the brain, and map that data to thoughts and intentions.
“We’re trying to ‘teach’ the computer to recognize patterns in blood flow, and guess what that person might be thinking,” said Chau, pictured below, left.
Before the computer can read your mind, it needs to be trained to understand how your brain works. Chau and his team have developed a set of training paradigms that ask the subject to perform a mental task, such as singing a song in your head, performing mental arithmetic or thinking of all the words you know that start with a given letter of the alphabet. These tasks induce patterns of blood flow that are generally identifiable and relatively similar across individuals.
The next step is for you to “send” blood to different part of your brain without performing the mental task.
“When people come into our lab, they are always sceptical — they don’t believe they can do it,” Chau said. “In our studies, we’ve shown that by the tenth session people can modulate blood flow in their brain without the task. They learn how it feels.”
They’ve also taught the computer to recognize a relaxed, wandering mind — not so simple, it turns out.
“The brain at rest is incredibly noisy,” he said.
Chau’s team works at the leading edge of both medicine and engineering, and welcomes fresh ideas and approaches from all fields.
“Collaboration is critical in our group,” he said. “I’ve had exceptional grad students from U of T — from IBBME, but also chemical, electrical, aerospace and mechanical. All of them bring such innovative approaches to biomedical challenges.”
Machine learning
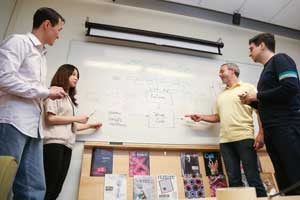
In the decade since the genome was sequenced in 2003, scientists, engineers and doctors have struggled to answer an all-consuming question: Which DNA mutations cause disease?
Professor Brendan Frey (ECE) and his team aim to revolutionize genomic medicine by applying advanced deep-learning computational techniques to unravel mysteries contained in the three-billion paired DNA molecules that make up the human genome.
In 2015, Frey and his collaborators founded Deep Genomics, the first company to combine the group’s decades of world-leading expertise with machine learning and genomic science.
“Our vision is to change the course of genomic medicine,” said Frey, the company’s president and CEO. “We’re inventing a new generation of deep learning technologies that can tell us what will happen within a cell when DNA is altered by natural mutations, therapies or even by deliberate gene editing.
In its first year, Deep Genomics secured $5 million in seed funding and has grown to 17 full-time employees. Its multidisciplinary scientific advisory board includes Yann Lecun, director of artificial intelligence research at Facebook, Stephen Scherer, director of the Centre for Applied Genomics and world-leading expert on autism spectrum disorder, and Anshul Kundaje, a professor of genetics and computer science at Stanford University.
Frey feels that collaboration is key to informing the company’s direction. The network he and his colleagues are building will see their technology reach across disciplines and industries to benefit real patients, on a global scale.
“At its core, Deep Genomics is a research-driven company,” Frey said. “We want to have good relationships with the academic community and we want to provide a computational framework that will help speed up academic discoveries, as well as advances in medicine, including screening, genetic testing, drug targeting, personalized medicine and more effective treatment.”